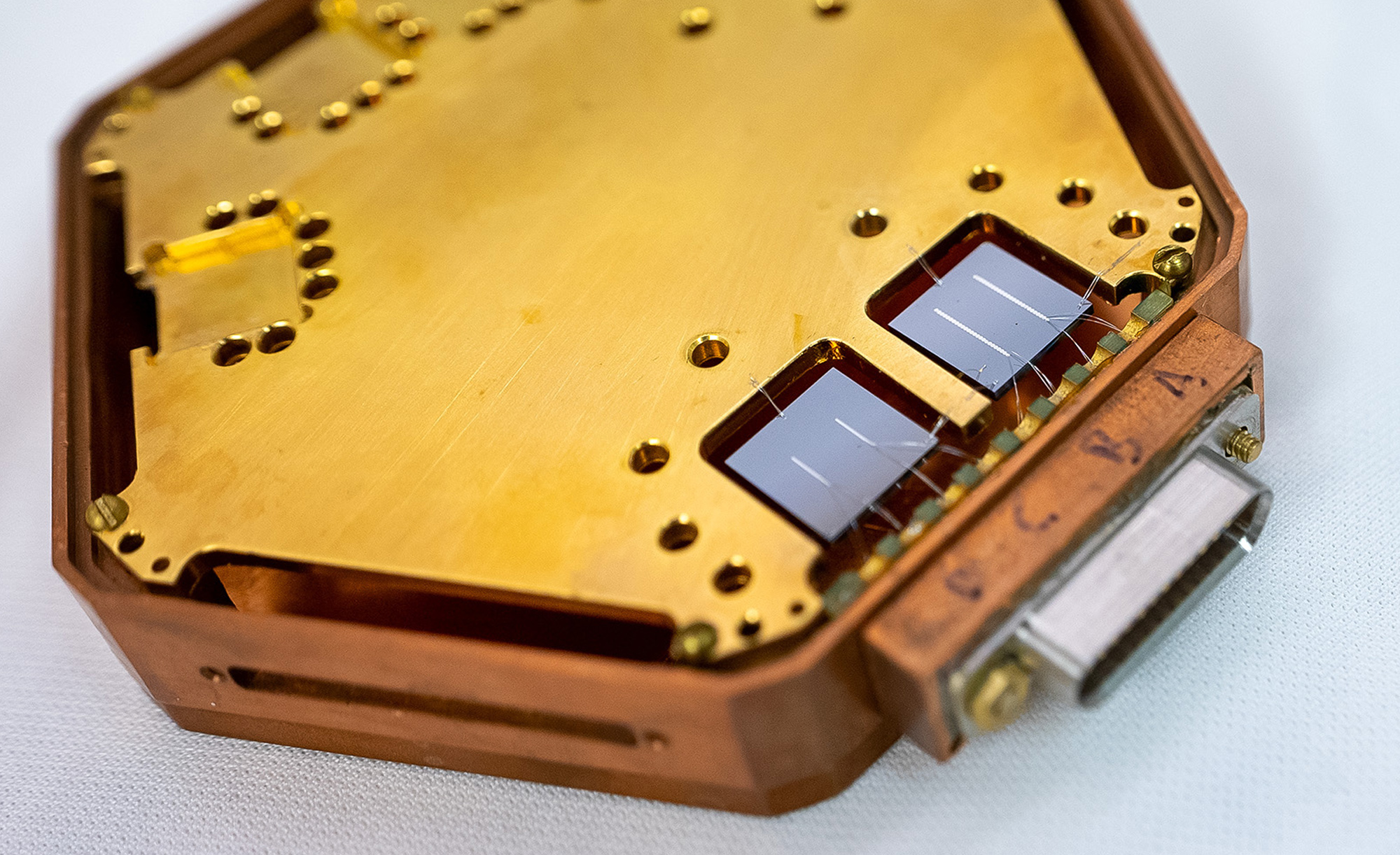
For decades, researchers across the world have been trying to directly detect dark matter: the missing mass in our universe. Now, there’s a new, super-sensitive detector on the case — and even though it’s still in the research and development phase, it’s already been able to search for kinds of dark matter that other detectors can’t reach.
Historically, most dark matter searches have hunted for two particular kinds of dark matter: ultra-light axions and heavier WIMPs (weakly interacting massive particles). But the new TESSERACT experiment searches in between these regimes, looking for low-mass dark matter that’s about a hundred to a thousand times lighter than a WIMP.
“It’s a kind of Goldilocks dark matter,” said Dan McKinsey, the project director for TESSERACT and a researcher at the U.S. Department of Energy’s (DOE) Lawrence Berkeley National Laboratory (Berkeley Lab), which leads the experiment. “There’s this untested window that gives us an opportunity for discovery. Our detector has the sensitivity, even at this early stage, that allows us to look for dark matter candidates no one has been able to look for before.”
The TESSERACT (Transition-Edge Sensors with Sub-EV Resolution And Cryogenic Targets) collaboration also includes researchers at Argonne National Laboratory, Caltech, Florida State University, IJCLab (Laboratoire de Physique des 2 Infinis Iréne Joliot-Curie), IP2I (Institut de Physique des 2 Infinis de Lyon), LPSC (Laboratoire de Physique Subatomique et de Cosmologie), Texas A&M University, UC Berkeley, the University of Massachusetts Amherst, the University of Zürich and QUP (the International Center for Quantum-field Measurement Systems for Studies of the Universe and Particles).
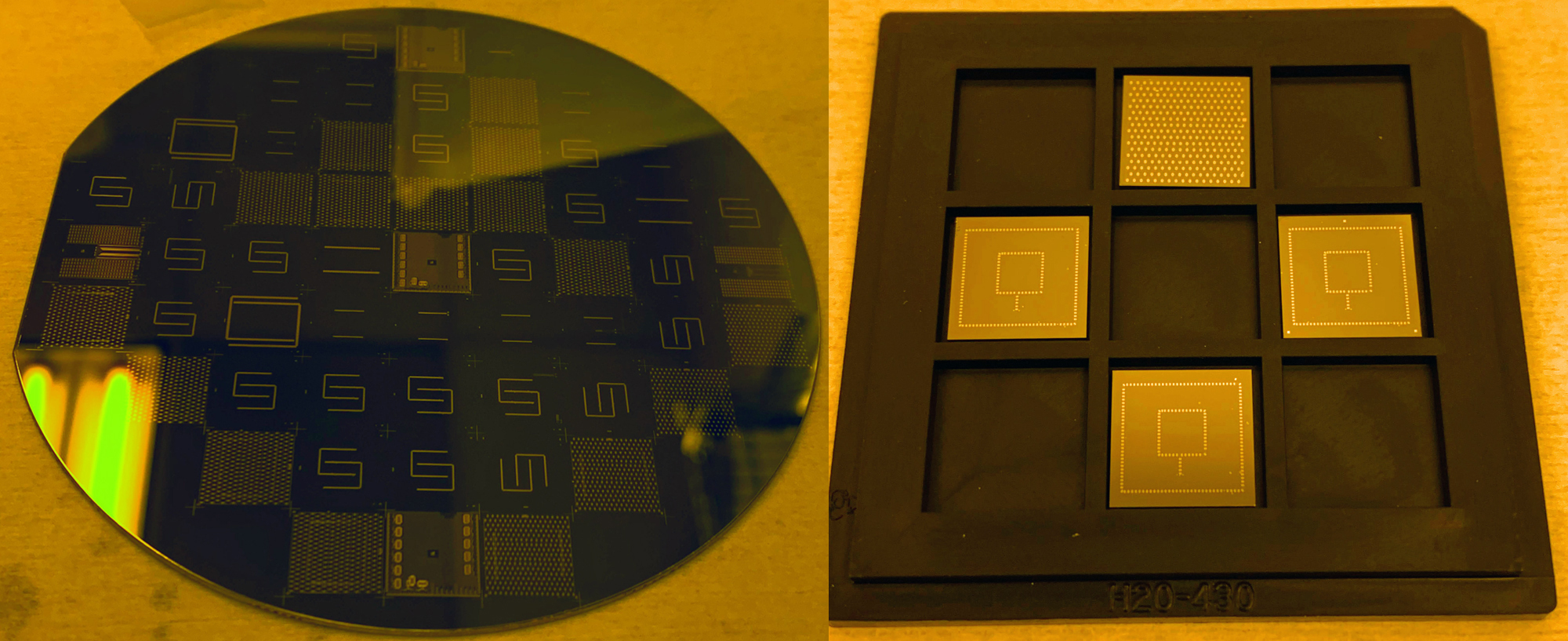
In a paper posted on March 5 to the online repository arXiv, TESSERACT researchers including Texas A&M physicist Rupak Mahapatra presented their first dark matter results, finding no evidence of low-mass dark matter between masses of 44 MeV/c2 and 87 MeV/c2 (where MeV is millions of electronvolts). For comparison, the mass of a proton is slightly less than 1,000 MeV/c2, and the most recent results by LZ, the world’s best WIMP experiment, searched for dark matter down to 9,000 MeV/c2. This is the first time any experiment has searched for nuclear recoil signals from dark matter with mass below 87 MeV/c2.
If researchers were to find dark matter in this new region, they’d need a new explanation for how it interacts. For example, while WIMPs interact through the weak force, low-mass dark matter could indicate an undiscovered fifth fundamental force at play.
Strength Through Super-Sensitivity
While many advanced dark matter experiments use huge volumes of detector material or giant magnets, TESSERACT’s power comes not from its size but its sensitivity. The new world-leading results came from carefully instrumented and shielded silicon chips that are one square centimeter, roughly a quarter of the size of a postcard stamp. These detectors were designed at UC Berkeley and fabricated at Texas A&M.
“We have been developing highly sensitive cryogenic detectors for the past 15 years for various experiments, including the SuperCDMS Dark Matter experiment at SNOLAB, Canada, the MINER reactor neutrino experiment at Texas A&M and several cutting-edge detector R&D projects funded by the Department of Energy along with seed funding provided by the Mitchell Institute,” said Mahapatra, a professor in the Texas A&M Department of Physics and Astronomy and member of the George P. and Cynthia Woods Mitchell Institute for Fundamental Physics and Astronomy since 2008. “That being said, the TESSERACT detector development was one of most challenging projects we have ever undertaken.”
Mahapatra, an international expert in high-energy particle physics and dark matter and a 2010 DOE Early Career Research Award recipient, credits his team’s decade-plus experience in developing cryogenic detectors for their ultimate success in fabricating R&D devices for TESSERACT during the past couple of years. The painstaking process — the latest endeavor in the Mahapatra Laboratory’s ongoing search for new interactions beyond what is known to exist in the Standard Model of particle physics — was led by research engineer Mark Platt, culminating in the science-ready detectors that have produced the collaboration’s world-leading results.
“We have been fortunate to have strong support from Texas A&M University through the Presidential Impact Fellowship, Chancellor’s EDGES Fellowship and the Mitchell-Heep endowed chair position as well as our annual Mitchell Institute funding,” said Mahapatra, who recently was officially appointed to the Mitchell-Heep Chair in Experimental High Energy Physics in recognition of his excellence in scholarship and contributions to his field. “Collectively, this support has helped us maintain a unique, fully dedicated semiconductor detector fabrication facility that produces sensors capable of searching for rare events without potential cross-contamination from a shared-use fabrication facility. We are excited about the highly sensitive searches for new physics enabled by our detector development expertise.”
Low Is The Way To Go
TESSERACT uses transition-edge sensors, a type of superconducting detector that operates at around 8 millikelvin (nearly negative 460 degrees Fahrenheit). Adding even a minuscule amount of heat — say, from a lightweight dark matter particle bumping into the chip and depositing some energy — can trip the sensor.
“We’ve been working to make the sensors very consistent and high-fidelity at very low temperatures,” said Vetri Velan, a Chamberlain Fellow at Berkeley lab and co-lead of the analysis. “The lower the transition temperature of the sensor, the better the noise performance and the better the sensitivity to dark matter. So, it’s all about how sensitive we can make these sensors.”
Researchers have been conducting R&D since 2020 to increase the sensitivity and reduce (or account for) potential sources of noise that might hide a dark matter signal. That includes finding the right recipe for manufacturing the sensors and reducing background vibration and electromagnetic interference in the dilution refrigerator that houses the silicon chip. The detector’s small size is also an asset; less area means fewer background interactions.
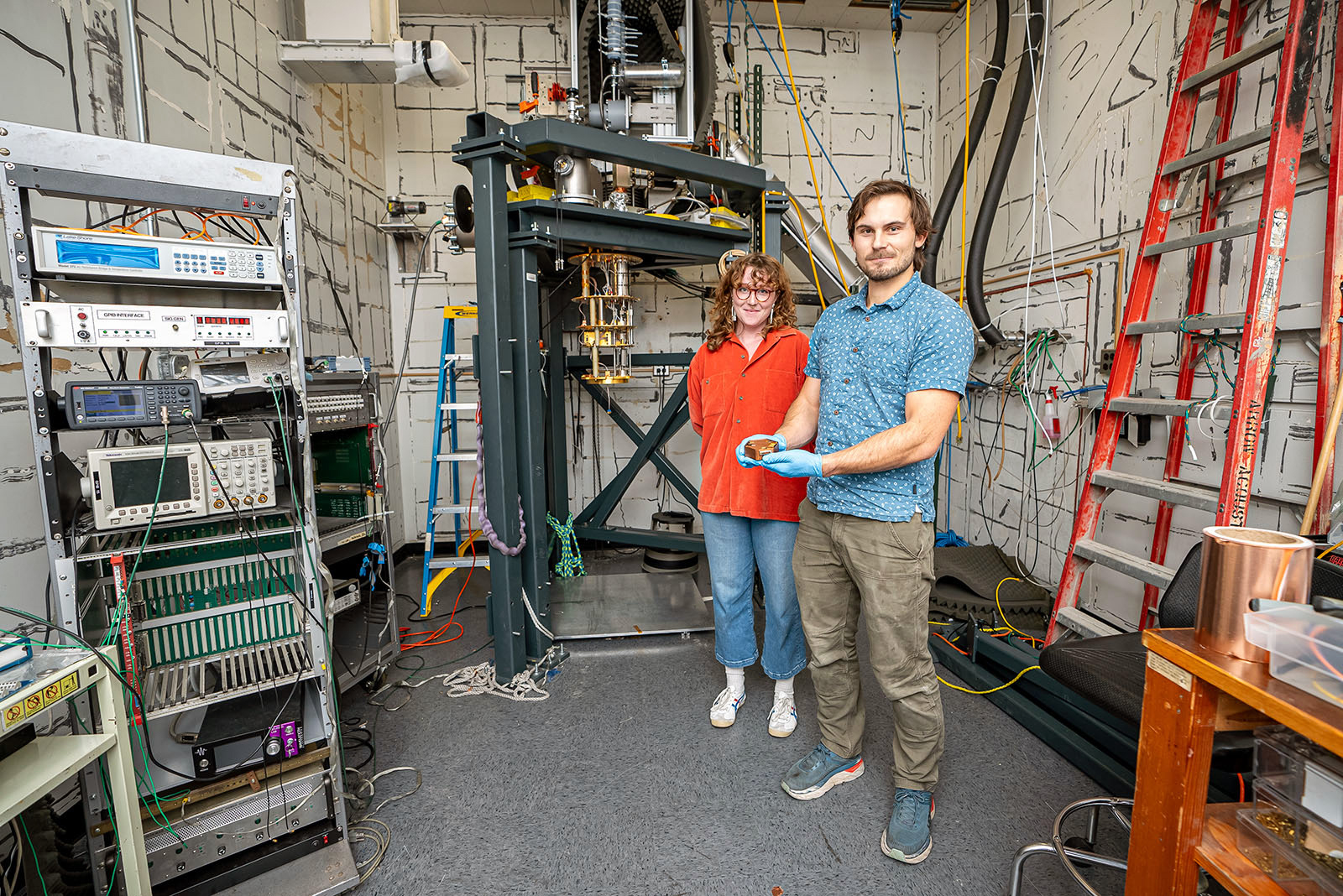
“The dedicated fabrication facility offers us exciting opportunities to modify standard semiconductor equipment to explore new techniques and hypotheses in detector design,” said Platt, an equipment engineer at Texas A&M and member of the Mahapatra Lab since 2009. “Coupled with my industry experience, we have developed an arsenal of equipment and skills during the past 15 years to produce superconducting detectors of various shapes, sizes and materials. We are capable of adjusting the fabrication process as the physics dictates, primarily limited only by our creativity. Because new ideas are often executed very quickly, it is an exciting time and place to create.”
TESSERACT addresses a problem common to sensitive detectors: a persistent but unexplained source of excess noise at low energies, the same region where dark matter might lurk. The experiment’s approach to manufacturing and shielding the detectors has reduced that background rate 30-fold.
“The devices that we are running are so quiet compared to pretty much any other device that’s ever been run,” said Michael Williams, a Chamberlain Fellow at Berkeley Lab and co-lead of the analysis. “And there’s a really large overlap between the work we’re doing on these devices and other quantum material science. As we improve these transition-edge sensors for ourselves, we can use the same engineering to make better qubits and quantum computers.”
An Experiment Designed To Change
Most dark matter experiments are placed deep underground, using thousands of feet of rock to help block out particles from space that can interact in the detector. With this first detector prototype, TESSERACT has already explored new spaces for dark matter from what is essentially Earth’s surface: the sub-basement of UC Berkeley’s Birge Hall (not far from the site of John Clauser’s Nobel Prize-winning experiment on photon entanglement that set the stage for our current quantum revolution).
But to further improve the detectors’ sensitivity, researchers plan to install the full experiment beneath 1,700 meters (5,600 feet) of rock in the deepest underground laboratory in Europe: France’s Modane Underground Laboratory. Construction is slated to begin in 2025, with the experiment coming online around 2029 and searching for dark matter with masses as low as 10 MeV/c2. The experiment will grow from its current footprint, roughly as big as a phone booth, to a six-foot cube.The devices that we are running are so quiet compared to pretty much any other device that’s ever been run. As we improve these transition-edge sensors for ourselves, we can use the same engineering to make better qubits and quantum computers.
The heart of TESSERACT is its transition-edge sensors, but researchers are also developing additional detector modules that improve their odds of spotting dark matter. HeRALD (Helium Roton Apparatus for Light Dark matter) will be the first time that a dark matter experiment uses superfluid helium and will incorporate TESSERACT's silicon chips as the detector's sensors. SPICE (Sub-ev Polar Interactions Cryogenic Experiment) will use single crystals of sapphire and gallium arsenide. And the science teams in France will contribute a sensor made of silicon and germanium. The different modules will give researchers unique ways to search for dark matter and test different theories.
The shielding that will surround the experiment is designed to come apart, making it possible for researchers to easily access TESSERACT’s components and switch things out in a matter of days. If HeRALD sees intriguing signs of dark matter, scientists can swap in SPICE and cross-check the result (or vice versa). In contrast, many advanced dark matter experiments are built like a ship in a bottle; it would take them months or years to open up and swap components, if it could be done at all.
Researchers are currently continuing to develop HeRALD and SPICE and testing new manufacturing processes to further improve the transition-edge sensors. “To get TESSERACT to the sensitivity we want, these detectors have to get even better, even though they’re already the best in the world,” Williams said.
Improved detectors and a subterranean home will let the experiment search for lower mass particles and increase the chance of detecting ultra-rare dark matter interactions with regular matter.
“This result is the first indication that we can open up this new regime of low-mass dark matter to experimental testing,” McKinsey said. “It’s a lot of fun to have a small experiment running in the basement that can test new ideas for dark matter. This is really just the opening salvo for TESSERACT. We expect to have many more results over the next decade.”
About Lawrence Berkeley National Laboratory
Lawrence Berkeley National Laboratory (Berkeley Lab) is committed to groundbreaking research focused on discovery science and solutions for abundant and reliable energy supplies. The lab’s expertise spans materials, chemistry, physics, biology, earth and environmental science, mathematics and computing. Researchers from around the world rely on the lab’s world-class scientific facilities for their own pioneering research. Founded in 1931 on the belief that the biggest problems are best addressed by teams, Berkeley Lab and its scientists have been recognized with 16 Nobel Prizes. Berkeley Lab is a multiprogram national laboratory managed by the University of California for the U.S. Department of Energy’s Office of Science, which is the nation’s single-largest supporter of basic research in the physical sciences. For more information, visit energy.gov/science.